Imagine you’re driving on the highway. Everyone is cruising along when all of a sudden, one car mistakenly breaks for no reason. This one small action could result in a multi-car pileup. Now think about that one small mistake on a global scale, but instead of driving, we’re talking about methane emissions–one of the most potent greenhouse gases driving climate change. Little changes can have massive outcomes.
Today, natural gas represents about 40% of the energy supply chain. As a result, increasing focus and scrutiny is on methane emissions; with studies like Miller et al. 2013, Alvarez et al. 2018, or Robertson et al. 2020 showing that emissions are highest in the upstream sector of the supply chain and significantly under-estimated.
But error bars are wide here: basin to basin variability is high due to geology. Operational practices significantly impact methane emissions, operator to operator variability is also significant.
I’ve seen this myself in the oilfield. A few years ago, I was with an operator in the DJ basin who had, on paper, gone above and beyond at their newest sites: no flares, electrification of compressors, elimination of tanks through funneling pipes directly to a central processing plant, and quarterly LDAR. But even among best-in-class operators, there is significant variability. For example, while this operator’s newer sites used up-to-date technology, they didn’t retrofit older sites with newer technology. This means old equipment (often needing repair, making noises, and chipping paint) remains in use alongside the latest technology. This variability is critical when it comes to thinking about environmental impact.
Now, let’s drill down into the actual math of how methane emissions can affect the overall carbon footprint of natural gas.
Let’s say that you buy gas from Producer A. Producer A has an upstream leak rate of 1%, and the rest of the supply chain– processing, transport, compressor stations along the way–experiences a leak rate of another 1%. (Note that I’m picking these numbers for mathematical convenience, but they’re also reasonably in line with analysis from the sources cited within. For example, Alvarez et al. 2018’s gathering through refining leak rate suggests .99%). Without actual measurements, we may, of course, be over-or under-estimating for a given producer, basin, or even facility.
Gas production is often measured by volume in SCF, a standard cubic foot, or MCF, a larger unit of 1000 SCF (temperature is implied here). Factoring in the 2% leak rate means that if you produce 1000 SCF, only 98% or 980 SCF makes it to market. Now, you may be thinking: is that gas entirely methane? That’s a great point, but the math will work out similarly. Say, for example, you have a gas composition that’s 84% methane, so you started with 1200 SCF of volume, of which approximately 1000 SCF is methane. Then you can start the analysis above again from 1000 SCF of methane, even though we started with mixed composition gas.
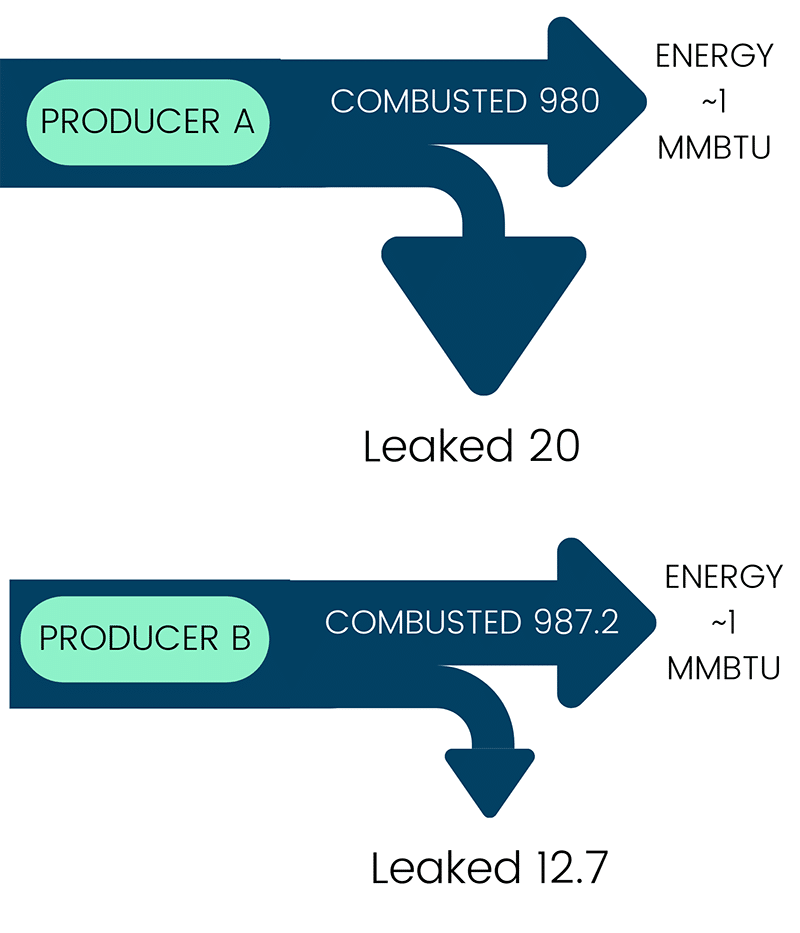
That 980 SCF of methane can be converted into energy. Let’s say that, like most gas in the US, this gas goes to the largest consumer of natural gas, an electric utility, and has an energy density of 1040 BTU/SCF. According to the EPA, burning or combusting natural gas produces 52.9 kg of carbon dioxide per MMBTU of energy generated. That means that if we combust this gas efficiently, our 980 SCF of gas has generated 53.9 kilograms of carbon and produced 1.02 MMBTU of energy.
If you’re lost with these units, consider this: A SCF is a standard cubic foot, or about the size of a breadbox, two shoe boxes stacked on top of each other, or one of those awkward decorative pillows on couches that are too small to provide any back support. A kilogram is about 2 pounds, which is about the size of a small bag of apples, a paperweight, or the smallest weights at your gym. For context, the average American generates about 16 tons or 16,000 kg of carbon emissions. MMBTU is an energy unit– one MMBTU is equal to 293-kilowatt hours; that’s the unit on your electric bill. For me, a Texas resident with AC and gas heating, this is the energy my home consumed in about a week during August 2020 or in the entire month of February 2020.
But in this accounting, the 20 SCF of gas was left out. That gas (assuming a specific volume of 1.474 cubic meters per kilogram at 70 degrees) is equivalent to 0.38 kg, or the weight of a really big tomato. While this may seem small in principle, the climate impact of this leaked gas is significant–it’s equivalent to 9.5 kg of carbon dioxide, or about the amount of emissions produced by one car in a year. This brings the total impact of that gas to 63.4 kg CO2e.
Comparing the 53.9 kg of carbon (from combustion) to the 9.5 kg of carbon (from leaks), we quickly see that the amount of carbon from emissions is a substantial percentage – about 15%. And those leaks didn’t even generate any energy–what a waste!
Now, consider Producer B, who’s made significant investments in technology, training, and operational practices. They’re located in a basin that produces gas near delivery to customers, and they’ve eliminated most of their emissions. Let’s say that these investments have resulted in an upstream emissions rate of 0.28% (this is a little under industry coalition ONE Future’s 2019 reported leak rate of 0.334%). Even if the rest of the supply chain has the same leak rate as Producer A, Producer B’s overall emissions rate of 1.28% is significantly lower than Producer A–by nearly 40%
That means that if a buyer could switch from Producer A to Producer B, they’d already score a big win, reducing methane emissions significantly.
So, what does that mean for the total carbon life cycle of this gas? Producer B produces 1000 SCF of gas, 987.2 SCF of which is delivered to their local power plant, producing 1.02 MMBTU of energy and generating 54.3 kg of emissions. This is pretty similar to our first scenario, except that we leaked less and, as such, generated slightly more energy. Along the way, 12.8 SCF of methane was lost into the atmosphere, generating 0.24 kg of carbon dioxide equivalent emissions. That brings the total carbon impact of this gas to 60 kg CO2e.
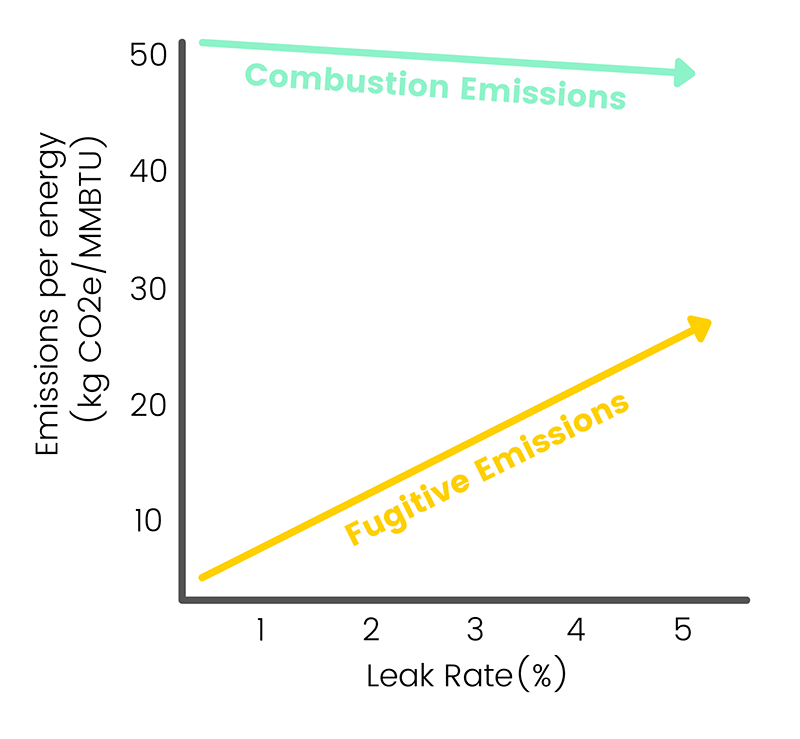
The difference between consuming energy generated from Producer A’s gas and Producer B’s gas is significant: approximately 3.3 kg CO2e or 14%. These are just two examples. To get an idea of how leak rate affects overall carbon footprint, we’ve graphed emissions as a function of leak rate (Figure X, left), which shows how sensitive emissions are to fugitive emissions as compared to combustion emissions.
How do these numbers add up at scale? Consider that an average utility can purchase 10,000- 100,000 MMBTUs or higher per day. Sourcing gas from Producer B over Producer A is impactful: making the switch at those purchase levels could save 33,000-330,000 kg of carbon dioxide equivalent every single day. To understand the impact yearly, we need to go up in units to metric tons (1000 kg): up to 120,450 tons of CO2e of carbon saved.
That’s equivalent to taking 26,000 cars off the road.
This demonstrates clearly why switching from one supplier to another, given adequate data, can generate fast and immediate emissions savings. The even better news is that these numbers are generated with reasonably conservative estimates–academic peer-reviewed studies and measurement campaigns sponsored by environmental groups suggest that leak rates may be even higher at up to 2.5% on average. Estimates in some basins like the Permian have been measured as underestimated by 1500% (Environ. Sci. Technol. 2020, 54, 21, 13926–13934). As leak rates grow, fugitive emissions represent a more significant proportion of natural gas’ overall carbon footprint- while at leak rates of 1%, fugitive emissions represent 14% of the emissions, at a leak rate of 3%, the fugitive emissions represent about a quarter of the lifecycle carbon emissions.
We’ve also used conservative numbers in our carbon accounting in this blog. Methane has a different impact on the climate than carbon dioxide because of its molecular structure and ability to absorb infrared radiation or heat. As a result, it cycles out of the atmosphere faster than carbon dioxide. This means that depending on the timeframe examined, methane’s impact on climate differs. For example, over a twenty-year period, methane’s impact on climate (what climate scientists would call the Global Warming Potential) is considered to be up to 86 times that of carbon dioxide [note- update to the new IPCC #s]. By contrast, by looking over a 100 year period, that number goes down to 25 times.
At Project Canary, we don’t take a position on how carbon accounting is accomplished. We only insist that it is done and rigorously, using actual measurements–not estimates.
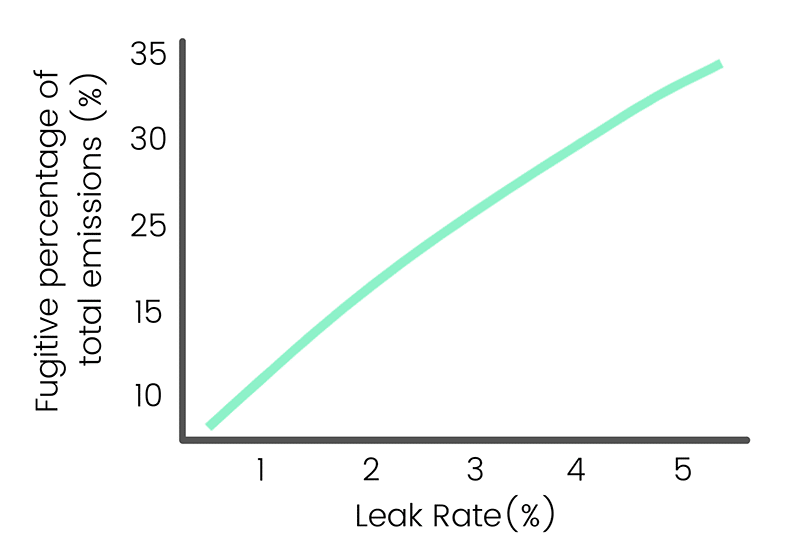
Nevertheless, these numbers bear out that the existence of methane leaks within the supply chain has a significant impact on the carbon footprint of the gas. Or, maybe not–but you’ll have to measure and then run the numbers to prove it.